Fourier Transform: Quantum Noise’s Newest Nemesis
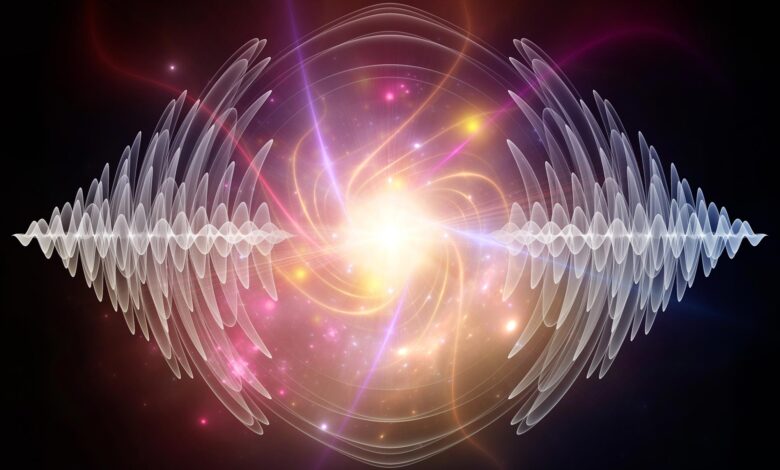
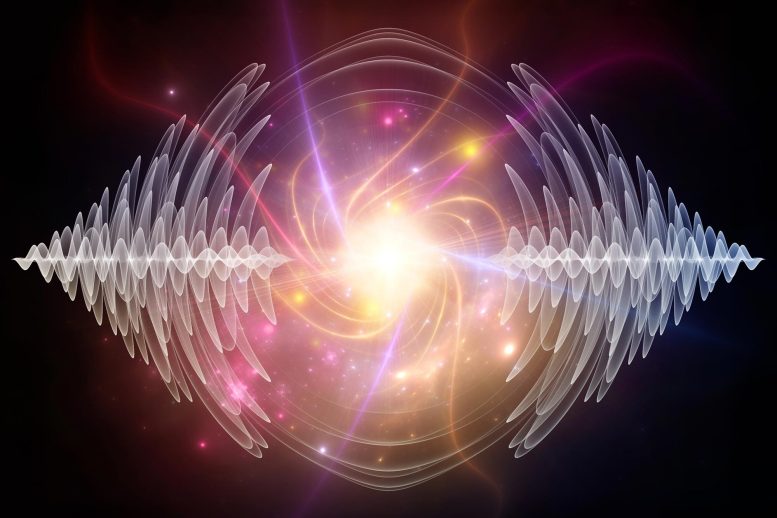
Researchers have developed a new method to better understand and mitigate quantum noise, which disrupts the operation of qubits. This innovation could enhance the reliability of quantum computing and sensing technologies by providing more detailed noise analysis and control.
A collaborative effort between physicists and chemists at the University of Colorado has led to the development of Fourier transform noise spectroscopy. This method simplifies the process of analyzing quantum noise, thereby facilitating advancements in quantum computing and sensing.
“Noise” is one of the biggest challenges in quantum technology and quantum sensing. These seemingly random environmental disturbances can disrupt the delicate quantum states of qubits, the fundamental units of quantum information.
Looking deeper at this issue, JILA Associate Fellow and University of Colorado Boulder Physics Assistant Professor Shuo Sun recently collaborated with Andrés Montoya-Castillo, assistant professor of Chemistry (also at CU Boulder), and his team to develop a new method for better understanding and controlling this noise, potentially paving the way for significant advancements in quantum computing, sensing, and control. Their new method, which uses a mathematical technique called a Fourier transform, was published recently in the journal npj Quantum Information.
The Problems With Noise
While some noise sources, like music, can be enjoyable, others, such as the sounds of traffic or a bustling city, can be distracting and even lead to health issues over time. At a microscopic level, noise can also pose significant challenges. Even the smallest fluctuations in room temperature or floor vibration, or the qubit system’s inherent instability, can disrupt a qubit’s coherence, causing it to lose its quantum state in a process known as decoherence.
“Lots of quantum technologies that people are very excited about, like quantum computers and quantum sensors, face a practical limitation, which is implementation on a larger scale with higher sensitivity,” explains CU Boulder Physics graduate student and co-first author of the paper, Nanako Shitara, who works in Montoya-Castillo’s group. “This is because these quantum systems, or qubits, are very sensitive to fluctuations in the surrounding fields, and they often interact with each other.”
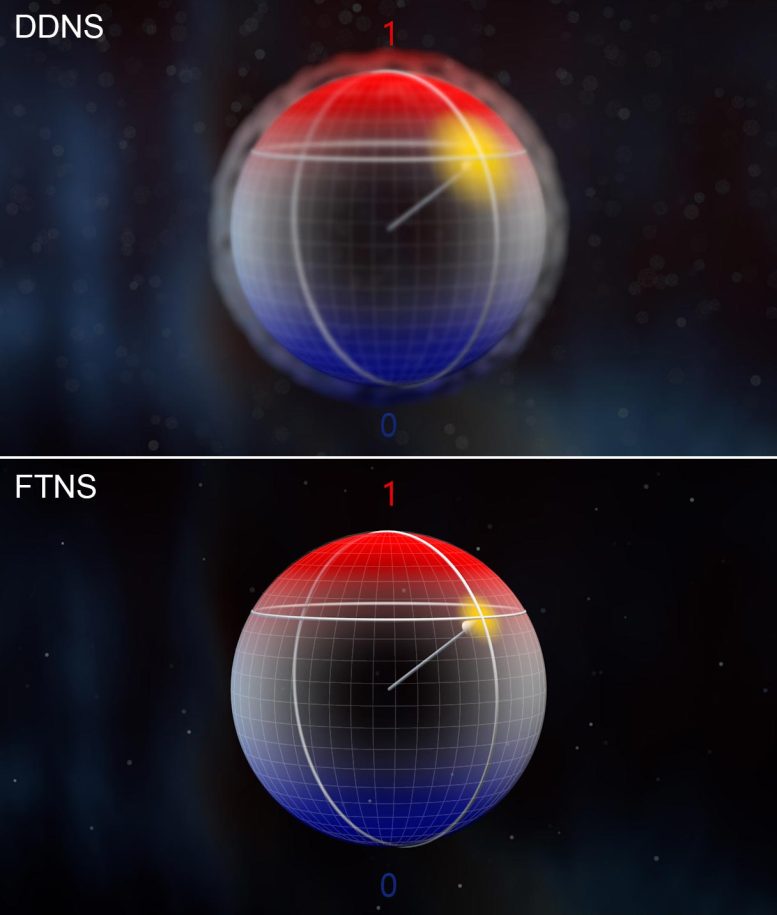
Comparison of two noise spectroscopy methods of qubit environments. The Sun group’s FTNS significantly outperforms current DDNS methods. Credit: Steven Burrows/Sun group
Not only does the noise affect the measurements of fragile systems like an ultra-precise quantum sensor, but it can also make the system less manageable.
Shitara elaborates, “The problem becomes a question of control: you want to control how a qubit reacts to certain kinds of noise. Basically, you want it to react to the right signals very well while it ignores other noise sources.”
Understanding the sources of this noise, and finding ways to mitigate them, is crucial for developing reliable quantum devices, such as quantum computers or sensors.
“Understanding the noise environment of a qubit is not only important for noise mitigation, but also serves as a valuable probe for materials,” Sun explains. “In the latter case, the qubit acts as a sensor, providing insights into the behavior of the surrounding material environment.”
Traditional Noise Characterization
To study and control this noise, scientists have traditionally used a method called dynamical decoupling noise spectroscopy (DDNS). This method involves applying precise pulses to the qubits and observing how they respond.
“Dynamical decoupling was originally, and still is, used for making the coherence times longer in qubits,” adds Shitara. “It turns out, that if you apply very short light pulses onto a qubit that is interacting with its environment, in some periodic manner…[it] helps the qubit’s coherence survive longer through some sort of effective decoupling.”
More recently, dynamical decoupling was repurposed as a noise spectroscopy method (hence DDNS) to measure and characterize the noise among the qubits. Though effective, DDNS is complex and requires applying a large number of almost instantaneous laser pulses. It also requires several assumptions about the underlying noise processes, making it cumbersome and less practical for widespread use.
Shitara elaborated that the DDNS method has minimum and maximum frequency limits for noise spectrum reconstruction due to physical constraints, potentially causing scientists to miss interesting phenomena. “You can see that the lowest frequency at which they reconstruct the spectrum can be actually quite high, depending on the implementation,” she adds.
Looking at the challenges of DDNS, Shitara, Sun, Montoya-Castillo, and CU Boulder postdoctoral researcher Arian Vezvaee proposed a new method that required fewer laser pulses and utilized a mathematical technique known as the Fourier transform.
Transforming Noise Maps
The new method, Fourier transform noise spectroscopy (FTNS), offers a straightforward, yet powerful, way to analyze the noise affecting qubits by focusing on the qubits’ coherence dynamics. Coherence measures how well a qubit maintains its quantum state, which is critical for its performance in quantum computations. These measurements are typically done through simple experiments like free induction decay (FID) or spin echo (SE), which start the qubit in a specific initial state and let its coherence decay freely over time, with zero or one intermediate pulses applied during the decay, respectively.
Once these time-based measurements are collected, the data is treated using the Fourier transform. This process is like breaking down a digital painting into its basic color spectrum, pixel by pixel, to understand the units of color that it’s made of. The units transform from pixels to color values through this process.
In this paper, the researchers used the Fourier transform to convert the time-domain data into frequency-domain data, effectively breaking down the complex signal into its constituent frequencies. By doing so, FTNS revealed the noise spectrum, showing which noise frequencies were present and how strong they were. The researchers found that the FTNS method also handled various types of noise, including complex noise patterns that were challenging for other methods like DDNS to decipher.
While a more streamlined method, FTNS has some limitations, like minimum and maximum frequency constraints and the need for high-resolution time and coherence measurements. However, the researchers demonstrated that these limitations are far less constraining than those of dynamical decoupling noise spectroscopy.
Sun and his team at JILA are now experimentally testing the FTNS method in nitrogen-vacancy centers, often found within synthetic diamonds that are used as qubits. Simultaneously, Joe Zadrozny, Associate Professor of Chemistry at Ohio State University, and his team are working to implement FTNS in molecular qubits and magnets.
“We are super excited about our method’s ability to reveal the frequency-resolved conversation between a qubit or sensor and its environment—and even more about the new opportunities it offers,” elaborated Montoya-Castillo. “From the sensing perspective, we are working to establish how FTNS can show hard-to-see physical processes near a sensor, whether this is a color center in a crystal, like nitrogen vacancies in diamond, trapped ions, or molecular magnets. This is an exciting frontier as quantum sensors may enable imaging of complex biological processes, like protein folding, with unprecedented detail and temporal resolution.”
Reference: “Fourier transform noise spectroscopy” by Arian Vezvaee, Nanako Shitara, Shuo Sun and Andrés Montoya-Castillo, 17 May 2024, npj Quantum Information.
DOI: 10.1038/s41534-024-00841-w
This research was supported by the National Science Foundation and the Sloan Research Fellowship.