Diamonds Grown Without High Pressure
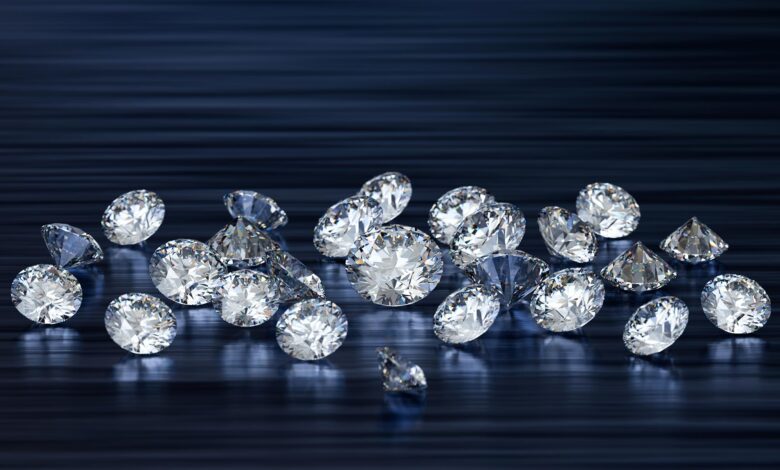
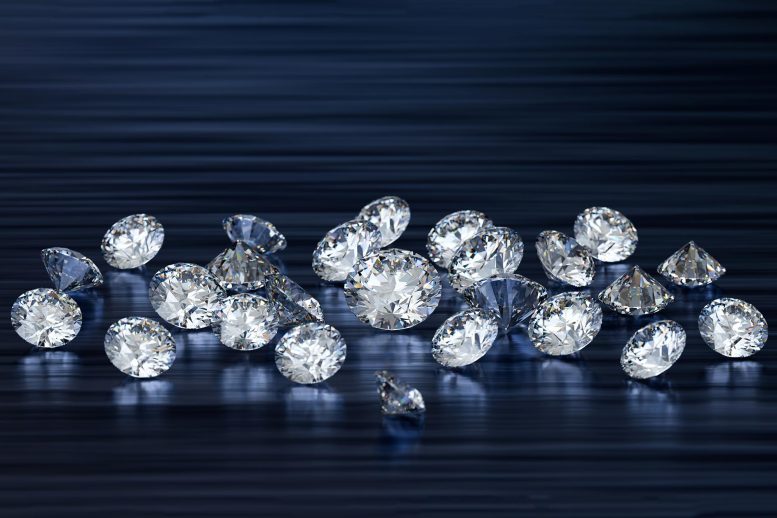
Researchers have pioneered a method to grow diamonds under low pressure and temperature, revolutionizing the traditional synthetic diamond production process and expanding possibilities for scientific and technological advancements.
Scientists have created a new liquid metal alloy system for producing diamonds under moderate conditions.
Did you know that 99% of synthetic diamonds are produced using high-pressure and high-temperature (HPHT) methods? The common belief is that diamonds can only be grown with liquid metal catalysts at pressures of 5-6 gigapascals (about 50,000-60,000 atmospheres) and temperatures between 1300-1600 °C. However, the size of diamonds produced through HPHT is typically limited to about one cubic centimeter due to the limitations of the process.
That is—achieving such high pressures can only be done at a relatively small length scale. Discovering alternative methods to make diamonds in liquid metal under milder conditions (particularly at lower pressure) is an intriguing basic science challenge that if achieved could revolutionize diamond manufacturing. Could the prevailing paradigm be challenged?
A team of researchers led by Director Rod Ruoff at the Center for Multidimensional Carbon Materials (CMCM) within the Institute for Basic Science (IBS), including graduate students at the Ulsan National Institute of Science and Technology (UNIST), have grown diamonds under conditions of 1 atmosphere pressure and at 1025 °C using a liquid metal alloy composed of gallium, iron, nickel, and silicon, thus breaking the existing paradigm. The discovery of this new growth method opens many possibilities for further basic science studies and for scaling up the growth of diamonds in new ways.
Enhancing Experimental Efficiency
Director Ruoff, who is also a UNIST Distinguished Professor notes, “This pioneering breakthrough was the result of human ingenuity, unremitting efforts, and the concerted cooperation of many collaborators.” Researchers led by Ruoff conducted a series of experiments, involving several hundred parameter adjustments and a variety of experimental approaches before they finally succeeded in growing diamonds using a ‘home-built’ cold-wall vacuum system.
Ruoff notes “We had been running our parametric studies in a large chamber (named RSR-A with an interior volume of 100 liters) and our search for parameters that would yield growth of diamond was slowed due to the time needed to pump out air (about 3 minutes), purge with inert gas (90 minutes), followed by pumping down again to vacuum level (3 minutes) so that the chamber could then be filled with 1 atmosphere pressure of quite pure hydrogen/methane mixture (again 90 minutes); that is over 3 hours before the experiment could be started! I asked Dr. Won Kyung SEONG to design & build a much smaller chamber to greatly reduce the time needed to start (and finish!) the experiment with the liquid metal exposed to the mixture of methane and hydrogen.” Seong adds, “Our new homebuilt system (named RSR-S, with an interior volume of only 9 liters) can be pumped out, purged, pumped out, and filled with methane/hydrogen mixture, in a total time of 15 minutes. Parametric studies were greatly accelerated, and this helped us discover the parameters for which diamond grows in the liquid metal!”
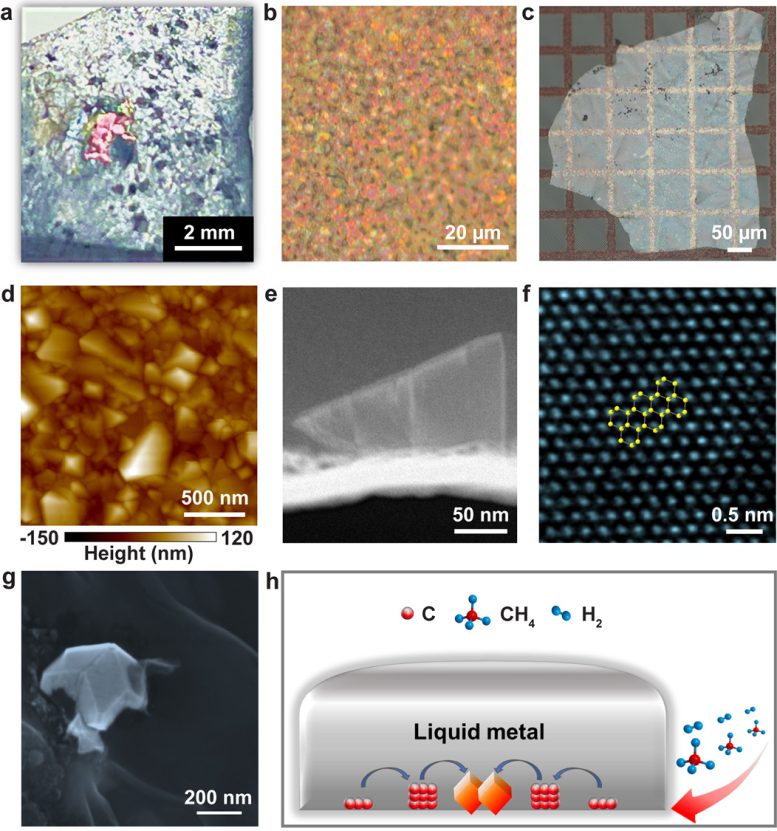
Growth of diamond in liquid metal alloy under 1 atmosphere pressure. (a) A photo showing the as-grown diamond on the solidified liquid metal surface. (b) An optical image of the as-grown continuous diamond film on the solidified liquid metal surface. (c) An optical image of the as-transferred diamond film on a Quantifoil holey amorphous carbon film coated Cu TEM grid. (d) An atomic force microscopy topographic image of the as-transferred diamond film on the Cu TEM grid. (e) A cross-section TEM image of an as-grown single diamond particle on the solidified liquid metal surface. (f) An atomic resolution TEM image of the as-grown diamond. (g) A scanning electron microscopy image showing a grown diamond (partially) submerged in the solidified liquid metal. (h) Scheme showing the diffusion of carbon that leads to the growth of diamond at the bottom surface of the liquid metal. Credit: Institute for Basic Science
The team discovered that diamond grows in the sub-surface of a liquid metal alloy consisting of a 77.75/11.00/11.00/0.25 mix (atomic percentages) of gallium/nickel/iron/silicon when exposed to methane and hydrogen under 1 atm pressure at ~1025 °C.
Yan GONG, UNIST graduate student and first author, explains “One day with the RSR-S system when I ran the experiment and then cooled down the graphite crucible to solidify the liquid metal, and removed the solidified liquid metal piece, I noticed a ‘rainbow pattern’ spread over a few millimeters on the bottom surface of this piece. We found out that the rainbow colors were due to diamonds! This allowed us to identify parameters that favored the reproducible growth of diamond.”
The initial formation occurs without the need for diamond or other seed particles commonly used in conventional HPHT and chemical vapor deposition synthesis methods. Once formed, the diamond particles merge to form a film, which can be easily detached and transferred to other substrates, for further studies and potential applications.
The synchrotron two-dimensional X-ray diffraction measurements confirmed that the synthesized diamond film has a very high purity of the diamond phase. Another intriguing aspect is the presence of silicon-vacancy color centers in the diamond structure, as an intense zero-phonon line at 738.5 nm in the photoluminescence spectrum excited by using a 532 nm laser was found.
Coauthor Dr. Meihui WANG notes, “This synthesized diamond with silicon-vacancy color centers may find applications in magnetic sensing and quantum computing.”
Mechanisms and Theoretical Insight
The research team delved deeply into possible mechanisms for diamonds to nucleate and grow under these new conditions. High-resolution transmission electron microscope (TEM) imaging on cross-sections of the samples showed about 30-40 nm thick amorphous subsurface region in the solidified liquid metal that was directly in contact with the diamonds. Coauthor Dr. Myeonggi CHOE notes, “Approximately 27 percent of atoms that were present at the top surface of this amorphous region were carbon atoms, with the carbon concentration decreasing with depth.”
Ruoff elaborates, “The presence of such a high concentration of carbon ‘dissolved’ in a gallium-rich alloy could be unexpected, as carbon is reported to be not soluble in gallium. This may explain why this region is amorphous—while all other regions of the solidified liquid metal are crystalline. This sub-surface region is where our diamonds nucleate and grow and we thus focused on it.”
Researchers exposed the Ga-Fe-Ni-Si liquid metal to the methane/hydrogen for short periods of time to try to understand the early growth stage—well prior to the formation of a continuous diamond film. They then analyzed the concentrations of carbon in the subsurface regions using time-of-flight secondary ion mass spectrometry depth profiling. After a 10-minute run, no diamond particles were evident but there were ~65 at% carbon atoms present in the region where the diamond typically grows. Diamond particles began to be found after a 15-minute run, and there was a lower subsurface C atom concentration of ~27 at%.
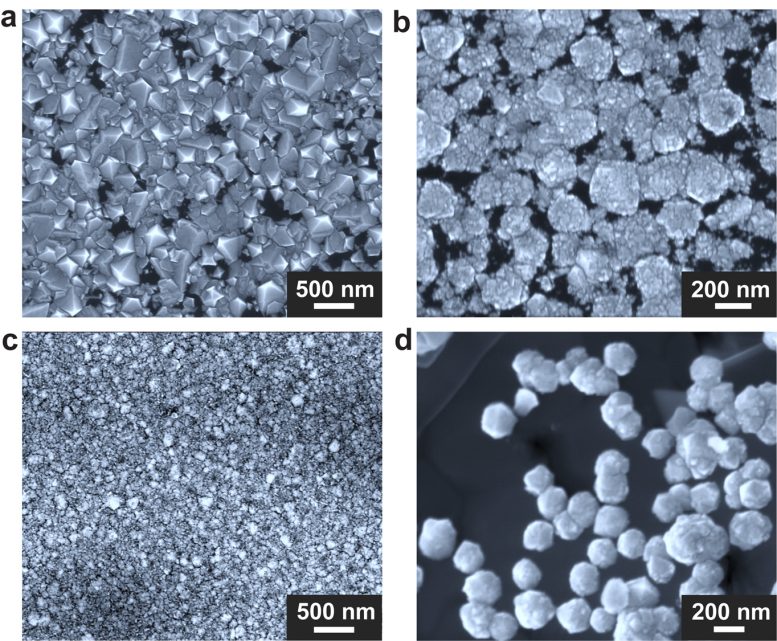
Diamonds of various morphologies as grown under different growth conditions. (a) Growth by using a liquid metal alloy of Ga/Ni/Fe/Si (77.75/11.00/11.00/0.25 at%) under methane/hydrogen (1/20 molar ratio). (b) Growth by using a liquid metal alloy of Ga/Ni/Fe/Si (77.50/11.00/11.00/0.50 at%) under methane/hydrogen (1/20 molar ratio). (c) Growth by using a liquid metal alloy of Ga/In/Ni/Fe/Si (38.88/38.87/7.33/14.67/0.25 at%) under methane/hydrogen (1/20 molar ratio). (d) Growth by using a liquid metal alloy of Ga/Ni/Fe/Si (77.75/11.00/11.00/0.25 at%) under methane/hydrogen (1/5 molar ratio). Credit: Institute for Basic Science
Ruoff explains, “The concentration of subsurface carbon atoms is so high at around 10 minutes that this time exposure is close to or at supersaturation, leading to the nucleation of diamonds either at 10 minutes or sometime between 10 and 15 minutes. The growth of diamond particles is expected to occur very rapidly after nucleation, at some time between about 10 minutes and 15 minutes.”
The temperature in 27 different locations in the liquid metal was measured with an attachment to the growth chamber having an array of nine thermocouples that was designed and built by Seong. The central region of the liquid metal was found to be at a lower temperature compared to the corners and sides of the chamber. It is thought that this temperature gradient is what drives carbon diffusion towards the central region, facilitating diamond growth.
The team also discovered that silicon plays a critical role in this new growth of diamond. The size of the grown diamonds becomes smaller and their density higher as the concentration of silicon in the alloy was increased from the optimal value. Diamonds could not be grown at all without the addition of silicon, which suggests that silicon may be involved in the initial nucleation of diamond.
This was supported by the various theoretical calculations conducted to uncover the factors that may be responsible for the growth of diamonds in this new liquid metal environment. Researchers found that silicon promotes the formation and stabilization of certain carbon clusters by predominantly forming sp3 bonds like carbon. It is thought that small carbon clusters containing Si atoms might serve as the ‘pre-nuclei’, which can then grow further to nucleate a diamond. It is predicted that the likely size range for an initial nucleus is around 20 to 50 C atoms.
Ruoff states, “Our discovery of nucleation and growth of diamond in this liquid metal is fascinating and offers many exciting opportunities for more basic science. We are now exploring when nucleation, and thus the rapid subsequent growth of diamond, happens. Also ‘temperature drop’ experiments where we first achieve supersaturation of carbon and other needed elements, followed by rapidly lowering the temperature to trigger nucleation— are some studies that seem promising to us.”
The team discovered their growth method offers significant flexibility in the composition of liquid metals. Researcher Dr. Da LUO remarks, “Our optimized growth was achieved using the gallium/nickel/iron/silicon liquid alloy. However, we also found that high-quality diamond can be grown by substituting nickel with cobalt or by replacing gallium with a gallium-indium mixture.”
Ruoff concludes, “Diamond might be grown in a wide variety of relatively low melting point liquid metal alloys such as containing one or more of indium, tin, lead, bismuth, gallium, and potentially antimony and tellurium—and including in the molten alloy other elements such as manganese, iron, nickel, cobalt and so on as catalysts, and others as dopants that yield color centers. And there is a wide range of carbon precursors available besides methane (various gases, and also solid carbons). New designs and methods for introducing carbon atoms and/or small carbon clusters into liquid metals for diamond growth will surely be important, and the creativity and technical ingenuity of the worldwide research community seem likely to me, based on our discovery, to rapidly lead to other related approaches and experimental configurations. There are numerous intriguing avenues to explore!”
Reference: “Growth of diamond in liquid metal at 1 atm pressure” by Yan Gong, Da Luo, Myeonggi Choe, Yongchul Kim, Babu Ram, Mohammad Zafari, Won Kyung Seong, Pavel Bakharev, Meihui Wang, In Kee Park, Seulyi Lee, Tae Joo Shin, Zonghoon Lee, Geunsik Lee and Rodney S. Ruoff, 24 April 2024, Nature.
DOI: 10.1038/s41586-024-07339-7