Atom Smasher Shatters Records With Heaviest Antimatter Discovery
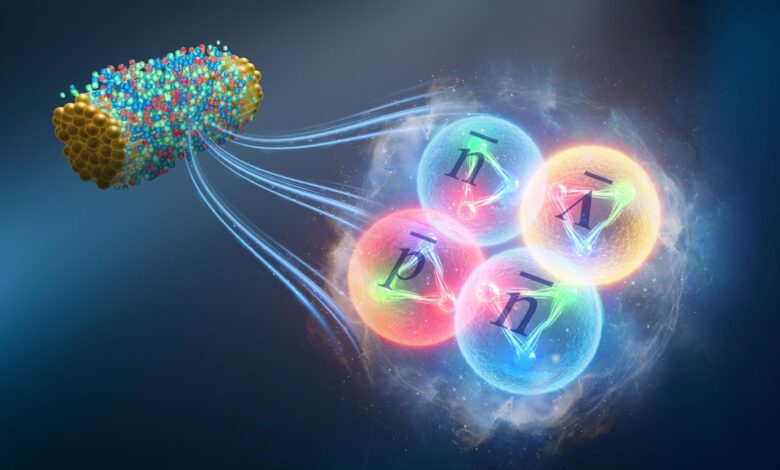
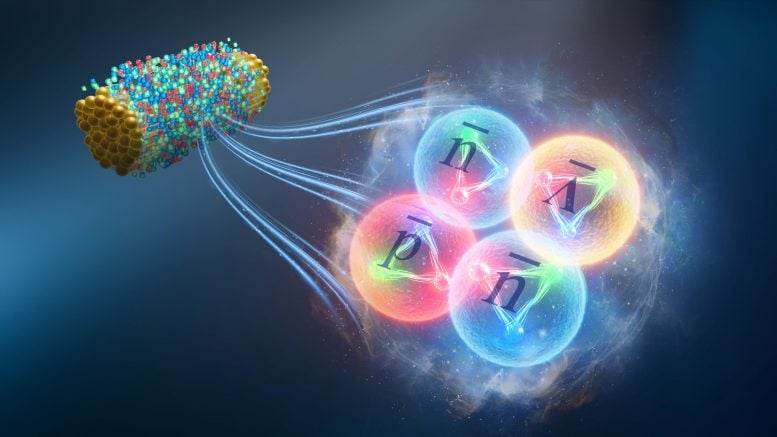
Scientists sifting through six billion particle smashups detect roughly 16 “antihyperhydrogen-4” particles, the heaviest antimatter nucleus discovered to date.
A groundbreaking discovery of the heaviest antimatter nucleus yet has been made at the RHIC, involving an antiproton, two antineutrons, and an antihyperon. This research aids in understanding why matter dominates the universe and confirms the fundamental properties of antimatter, suggesting no significant differences in particle decay rates compared to matter.
Discovery of a New Antimatter Nucleus
Scientists studying the tracks of particles streaming from six billion collisions of atomic nuclei at the Relativistic Heavy Ion Collider (RHIC) — an “atom smasher” that recreates the conditions of the early universe — have discovered a new kind of antimatter nucleus, the heaviest ever detected. Composed of four antimatter particles — an antiproton, two antineutrons, and one antihyperon — these exotic antinuclei are known as antihyperhydrogen-4.
Members of RHIC’s STAR Collaboration made the discovery by using their house-sized particle detector to analyze the details of the collision debris. They report their results today (August 21) in the journal Nature and explain how they’ve already used these exotic antiparticles to look for differences between matter and antimatter.
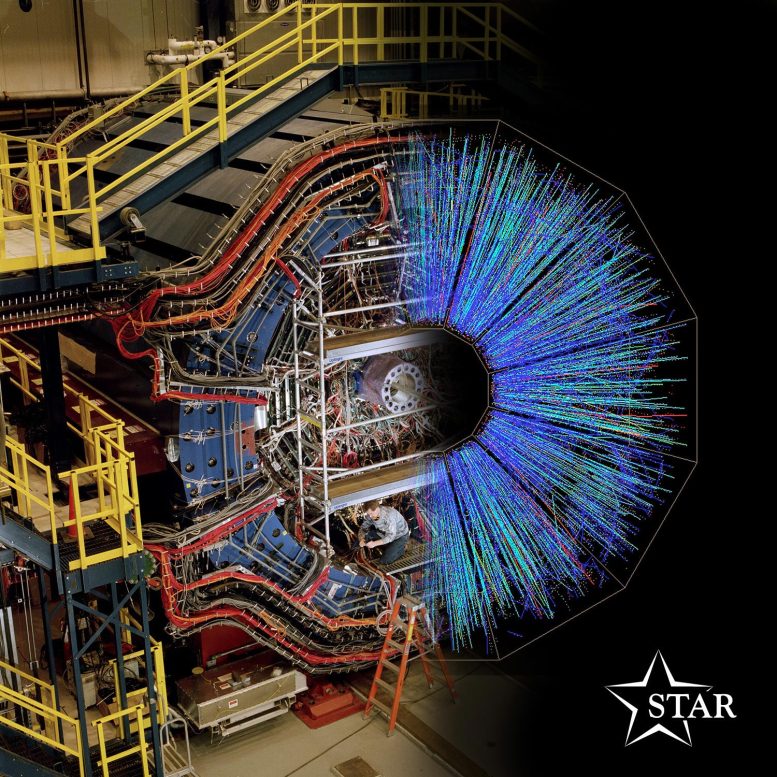
Understanding Matter-Antimatter Asymmetry
“Our physics knowledge about matter and antimatter is that, except for having opposite electric charges, antimatter has the same properties as matter — same mass, same lifetime before decaying, and same interactions,” said STAR collaborator Junlin Wu, a graduate student at the Joint Department for Nuclear Physics, Lanzhou University and Institute of Modern Physics, China. But the reality is that our universe is made of matter rather than antimatter, even though both are believed to have been created in equal amounts at the time of the Big Bang some 14 billion years ago.
“Why our universe is dominated by matter is still a question, and we don’t know the full answer,” Wu said.
RHIC, a U.S. Department of Energy (DOE) Office of Science user facility for nuclear physics research at DOE’s Brookhaven National Laboratory, is a good place to study antimatter. Its collisions of heavy ions — atomic nuclei that have been stripped of their electrons and accelerated close to the speed of light — melt the boundaries of the ions’ individual protons and neutrons. The energy deposited in the resulting soup of free quarks and gluons, visible matter’s most fundamental building blocks, generates thousands of new particles. And like the early universe, RHIC makes matter and antimatter in nearly equal amounts. Comparing characteristics of matter and antimatter particles generated in these particle smashups might offer clues to some asymmetry that tipped the balance to favor the existence of matter in today’s world.
Detecting Heavy Antimatter
“To study the matter-antimatter asymmetry, the first step is to discover new antimatter particles,” said STAR physicist Hao Qiu, Wu’s advisor at IMP. “That’s the basic logic behind this study.”
STAR physicists had previously observed nuclei made of antimatter created in RHIC collisions. In 2010, they detected the antihypertriton. This was the first instance of an antimatter nucleus containing a hyperon, which is a particle containing at least one “strange” quark rather than just the lighter “up” and “down” quarks that make up ordinary protons and neutrons. Then, just a year later, STAR physicists toppled that heavyweight antimatter record by detecting the antimatter equivalent of the helium nucleus: antihelium-4.
A more recent analysis suggested that antihyperhydrogen-4 might also be within reach. But detecting this unstable antihypernucleus — where the addition of an antihyperon (specifically an antilambda particle) in place of one of the protons in antihelium would edge out the heavyweight record holder once again — would be a rare event. It would require all four components — one antiproton, two antineutrons, and one antilambda — to be emitted from the quark-gluon soup generated in RHIC collisions in just the right place, headed in the same direction, and at the right time to clump together into a temporarily bound state.
“It is only by chance that you have these four constituent particles emerge from the RHIC collisions close enough together that they can combine to form this antihypernucleus,” said Brookhaven Lab physicist Lijuan Ruan, one of two co-spokespersons for the STAR Collaboration.
Needle in a “Pi” Stack
To find antihyperhydrogen-4, the STAR physicists looked at the tracks of the particles this unstable antihypernucleus decays into. One of those decay products is the previously detected antihelium-4 nucleus; the other is a simple positively charged particle called a pion (pi+).
“Since antihelium-4 was already discovered in STAR, we used the same method used previously to pick up those events and then reconstructed them with pi+ tracks to find these particles,” Wu said.
By reconstruct, he means retracing the trajectories of the antihelium-4 and pi+ particles to see if they emerged from a single point. But RHIC smashups produce a lot of pions. And to find the rare antihypernuclei, the scientists were sifting through billions of collision events! Each antihelium-4 emerging from a collision could be paired with hundreds or even 1,000 pi+ particles.
“The key was to find the ones where the two particle tracks have a crossing point, or decay vertex, with particular characteristics,” Ruan said. That is, the decay vertex has to be far enough from the collision point that the two particles could have originated from the decay of an antihypernucleus formed just after the collision from particles initially generated in the fireball.
The STAR team worked hard to rule out the background of all the other potential decay pair partners. In the end, their analysis turned up 22 candidate events with an estimated background count of 6.4.
“That means around six of the ones that look like decays from antihyperhydrogen-4 may just be random noise,” said Emilie Duckworth, a doctoral student at Kent State University whose role was to ensure that the computer code used to sift through all those events and pick out the signals was written properly.
Subtracting that background from 22 gives the physicists confidence they’ve detected about 16 actual antihyperhydrogen-4 nuclei.
Matter-Antimatter Comparison
The result was significant enough for the STAR team to do some direct matter-antimatter comparisons.
They compared the lifetime of antihyperhydrogen-4 with that of hyperhydrogen-4, which is made of the ordinary-matter varieties of the same building blocks. They also compared lifetimes for another matter-antimatter pair: the antihypertriton and the hypertriton.
Neither showed a significant difference, which did not surprise the scientists.
The experiments, they explained, were a test of a particularly strong form of symmetry. Physicists generally agree that a violation of this symmetry would be extremely rare and will not hold the answer to the matter-antimatter imbalance in the universe.
“If we were to see a violation of [this particular] symmetry, basically we’d have to throw a lot of what we know about physics out the window,” Duckworth said.
So, in this case, it was sort of comforting that the symmetry still works. The team agreed the results further confirmed that physicists’ models are correct and are “a great step forward in the experimental research on antimatter.”
The next step will be to measure the mass difference between the particles and antiparticles, which Duckworth, who was selected in 2022 to receive funding from the DOE Office of Science Graduate Student Research program, is pursuing.
Reference: “Observation of the Antimatter Hypernucleus 4/(anti)Λ(anti)H” 21 August 2024, Nature.
DOI: 10.1038/s41586-024-07823-0
This work was supported by the DOE Office of Science, the U.S. National Science Foundation, and a range of international agencies and organizations listed in the scientific paper. The researchers made use of computing resources in the Scientific Data and Computing Center at Brookhaven Lab, the National Energy Research Scientific Computing Center (NERSC) at DOE’s Lawrence Berkeley National Laboratory, and the Open Science Grid consortium. NERSC is another DOE Office of Science user facility.
Source link